Normally on “Mining and Refining,” we concentrate on the actual material that’s mined and refined. We’ve covered everything from copper to tungsten, with side trips to more unusual materials like sulfur and helium. The idea is to shine a spotlight on the geology and chemistry of the material while concentrating on the different technologies needed to exploit often very rare or low-concentration deposits and bring them to market.
This time, though, we’re going to take a look at not a specific resource, but a technique: fracking. Hydraulic fracturing is very much in the news lately for its potential environmental impact, both in terms of its immediate effects on groundwater quality and for its perpetuation of our dependence on fossil fuels. Understanding what fracking is and how it works is key to being able to assess the risks and benefits of its use. There’s also the fact that like many engineering processes carried out on a massive scale, there are a lot of interesting things going on with fracking that are worth exploring in their own right.
Fossil Mud
Although hydraulic fracturing has been used since at least the 1940s to stimulate production in oil and gas wells and is used in all kinds of well drilled into multiple rock types, fracking is most strongly associated these days with the development of oil and natural gas deposits in shale. Shale is a sedimentary rock formed from ancient muds made from fine grains of clay and silt. These are some of the finest-grained materials possible, with grains ranging from 62 microns in diameter down to less than a micron. Grains that fine only settle out of suspension very slowly, and tend to do so only where there are no currents.
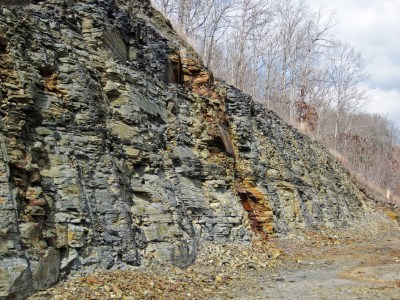
The breakup of Pangea during the Cretaceous period provided much of the economically important shale formations in today’s eastern United States, like the Marcellus formation that stretches from New York state into Ohio and down almost to Tennesee. The warm, calm waters of the newly forming Atlantic Ocean formed the perfect place for clay- and silt-laden runoff to accumulate and settle, eventually forming the shale formation.
Shale is often associated with oil and natural gas because the conditions that favor its formation also favor hydrocarbon creation. The warm, still Cretaceous waters were perfect for phytoplankton and algal growth, and when those organisms died they rained down along with the silt and clay grains to the low-oxygen environment at the bottom. Layer upon layer built up slowly over the millennia, but instead of decomposing as they would have in an oxygen-rich environment, the reducing conditions slowly transformed the biomass into kerogen, or solid deposits of hydrocarbons. With the addition of heat and pressure, the hydrocarbons in kerogen were cooked into oil and natural gas.
In some cases, the tight grain structure of shale acts as an impermeable barrier to keep oil and gas generated in lower layers from floating up, forming underground deposits of liquid and gas. In other cases, kerogens are transformed into oil or natural gas right within the shale, trapped within its pores. Under enough pressure, gas can even dissolve right into the shale matrix itself, to be released only when the pressure in the rock is relieved.
Horizontal Boring
While getting at these sequestered oil and gas deposits requires more than just drilling a hole in the ground, fracking starts with exactly that. Traditional well-drilling techniques, where a rotary table rig using lengths of drill pipe spins a drill bit into rock layers underground while pumping a slurry called drilling mud down the bore to cool and lubricate the bit, are used to start the well. The initial bore proceeds straight down until it passes through the lowest aquifer in the region, at which point the entire bore is lined with a steel pipe casing. The casing is filled with cementitious grout that’s forced out of the bottom of the casing by a plug inserted at the surface and pressed down by the drilling rig. This squeezes the grout between the outside of the casing and the borehole and back up to the surface, sealing it off from the water-bearing layers it passes through and serving as a foundation for equipment that will eventually be added to the wellhead, such as blow-out preventers.
Once the well is sealed off, vertical boring continues until the kickoff point, where the bore transitions from vertical to horizontal. Because the target shale seam is relatively thin — often only 50 to 300 feet (15 to 100 meters) thick — drilling a vertical bore through it would only expose a small amount of surface area. Fracking is all about increasing surface area and connecting as many pores in the shale to the bore; drilling horizontally within the shale seam makes that possible. Geologists and mining engineers determine the kickoff point based on seismic surveys and drilling logs from other wells in the area and calculate the radius needed to put the bore in the middle of the seam. Given that the drill string can only turn by a few degrees at most, the radius tends to be huge — often hundreds of meters.
Directional drilling has been used since the 1920s, often to steal oil from other claims, and so many techniques have been developed for changing the direction of a drill string deep underground. One of the most common methods used in fracking wells is the mud motor. Powered by drilling mud pumped down the drill pipe and forced between a helical stator and rotor, the mud motor can spin the drill bit at 60 to 100 RPM. When boring a traditional vertical well, the mud motor can be used in addition to spinning the entire drill string, to achieve a higher rate of penetration. The mud motor can also power the bit with the drill string locked in place, and by adding angled spacers between the mud motor and the drill string, the bit can begin drilling at a shallow angle, generally just a few degrees off vertical. The drill string is flexible enough to bend and follow the mud motor on its path to intersect the shale seam. The azimuth of the bore can be changed, too, by rotating the drill string so the bit heads off in a slightly different direction. Some tools allow the bend in the motor to be changed without pulling the entire drill string up, which represents significant savings.
Determining where the drill bit is under miles of rock is the job of downhole tools like the measurement while drilling (MWD) tool. These battery-powered tools vary in what they can measure, but typically include temperature and pressure sensors and inertial measuring units (IMU) to determine the angle of the bit. Some MWD tools also include magnetometers for orientation to Earth’s magnetic field. Transmitting data back to the surface from the MWD can be a problem, and while more use is being made of electrical and fiber optic connections these days, many MWDs use the drilling mud itself as a physical transport medium. Mud telemetry uses pressure waves set up in the column of drilling mud to send data back up to pressure transducers on the surface. Data rates are low; 40 bps at best, dropping off sharply with increasing distance. Mud telemetry is also hampered by any gas dissolved in the drilling mud, which strongly attenuates the signal.
Let The Fracking Begin
Once the horizontal borehole is placed in the shale seam, a steel casing is placed in the bore and grouted with cement. At this point, the bore is completely isolated from the surrounding rock and needs to be perforated. This is accomplished with a perforating gun, a length of pipe studded with small shaped charges. The perforating gun is prepared on the surface by pyrotechnicians who place the charges into the gun and connect them together with detonating cord. The gun is lowered into the bore and placed at the very end of the horizontal section, called the toe. When the charges are detonated, they form highly energetic jets of fluidized metal that lance through the casing and grout and into the surrounding shale. Penetration depth and width depend on the specific shaped charge used but can extend up to half a meter into the surrounding rock.
Perforation can also be accomplished non-explosively, using a tool that directs jets of high-pressure abrasive-charged fluid through ports in its sides. It’s not too far removed from water jet cutting, and can cut right through the steel and cement casing and penetrate well into the surrounding shale. The advantage to this type of perforation is that it can be built into a single multipurpose tool which can
Once the bore has been perforated, fracturing can occur. The principle is simple: an incompressible fluid is pumped into the borehole under great pressure. The fluid leaves the borehole and enters the perforations, cracking the rock and enlarging the original perforations. The cracks can extend many meters from the original borehole into the rock, exposing vastly more surface area of the rock to the borehole.
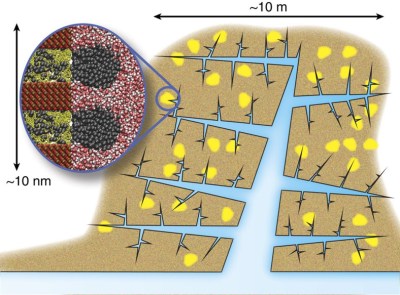
The pressure needed to hydraulically fracture solid rock perhaps a mile or more below the surface can be tremendous — up to 15,000 pounds per square inch (100 MPa). In addition to the high pressure, the fracking fluid must be pumped at extremely high volumes, up to 10 cu ft/s (265 lps). The overall volume of material needed is impressive, too — a 6″ borehole that’s 10,000 feet long would take almost 15,000 gallons of fluid to fill alone. Add in the volume of fluid needed to fill the fractures and that could easily exceed 5 million gallons.
Fracking fluid is a slurry made mostly from water and sand. The sand serves as a proppant, which keeps the tiny microfractures from collapsing after fracking pressure is released. Fracking fluid also contains a fraction of a percent of various chemical additives, mostly to form a gel that effectively transfers the hydraulic force while keeping the proppant suspended. Guar gum, a water-soluble polysaccharide extracted from guar beans, is often used to create the gel. Fracking gels are sometimes broken down after a while to clear the fractures and allow freer flow; a combination of acids and enzymes is usually used for this job.
Once fracturing is complete, the fracking fluid is removed from the borehole. It’s impossible to recover all the fluid; sometimes as much as 50% is recovered, but often as little as 5% can be pumped back to the surface. Once a section of the borehole has been fractured, it’s sealed off from the rest of the well by an isolating plug placed upstream of the freshly fracked section. The entire process — perforating, fracking, recovery, isolation — is repeated up the borehole until the entire horizontal bore is fracked. The isolating plugs are then bored out, and the well can begin production.